Videos
Loading the player ...
- Offer Profile
- The goal of the Biomimetic
Millisystems Lab is to harness features of animal manipulation, locomotion,
sensing, actuation, mechanics, dynamics, and control strategies to radically
improve millirobot capabilities. Research in the lab ranges from fundamental
understanding of mechanical principles to novel fabrication techniques to
system integration of autonomous millirobots. The lab works closely with
biologists to develop models of function which can be tested on engineered
and natural systems. The lab's current research is centered on all-terrain
crawling using nanostructured adhesives and bioinspired flight.
Product Portfolio
Ambulating Robots
-
Why legged robots?
Legged systems provide some key performance advantages compared to wheeled systems. In a legged system the feet are not continually in contact with the ground, whereas wheels require a continuous path of support. This enables legged organisms and robots to traverse challenging terrain. Some legged systems (biological and man-made) can overcome obstacles that are more than three times taller than the hip height of the system while wheels are limited to obstacles no higher than one radius. Finally, and perhaps most interesting is the dynamic behavior of legged organisms seen in nature. Many legged animals exhibit dynamically, self-stabilizing behavior. That is, the passive mechanical properties of the systems are tuned to naturally reject disturbances which might otherwise cause unstable behavior in the system.
Recent Results
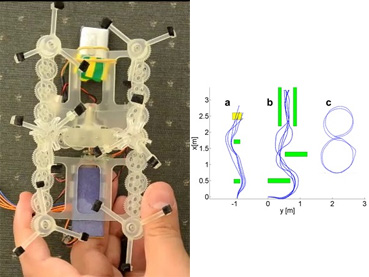
1STAR: 1 actuator STeerAble Robot
-
(June 2014)
We present a novel dynamic gait to control in-plane locomotion (forward, back, clockwise and counter clockwise rotations) of a compliant legged hexapedal robot using a single actuator. The gait exploits the compliance disparity between alternate stance tripods, to generate rotation by controlling the acceleration of the robot. Zarrouk and Fearing ( IEEE ICRA June 2014)
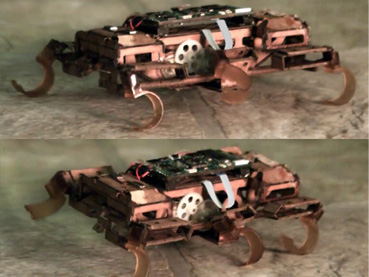
Roll oscillation modulated turning
-
(June 2014)
A new dynamic turning mode in legged robots is demonstrated, which uses a phase-locked gait to excite coupled height and roll oscillations. A modified robot with enhanced roll oscillations turned at 206 deg/sec at a speed of 0.4 m/s. Haldane and Fearing
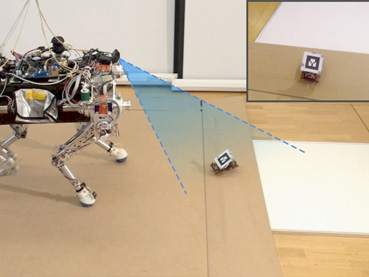
Detection of Slippery Terrain with Picket Robot
-
(June 2014)
Experiments conducted with StarlETH (ASL, ETH Zurich) and UCB VelociRoACH using joint localization detected slippery terrain with 92% accuracy. Haldane et al. ( IEEE ICRA June 2014)
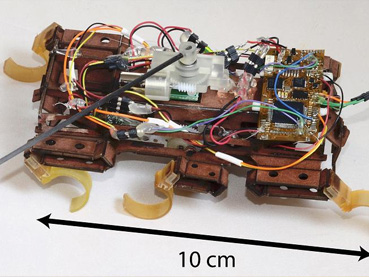
Aerodynamic Steering SailRoACH
-
SailRoACH uses aerodynamic forces on its tail to turn, achieving at 1.2 meter radius turn while running at 1.6 meters per second. Scaling laws work favorably for this turning mode for fast running small robots. Kohut et al. (IEEE IROS Nov. 2013)
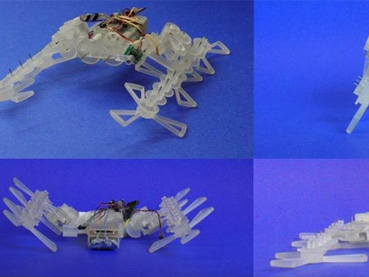
A Sprawl Tuned Autonomous Robot
-
(Feb. 2013)
This robot has a variable leg sprawl angle in the transverse plane to adapt its stiffness, height, and leg-to-surface contact angle. Contact angle and normal contact forces are substantially reduced when the sprawl angle is low, and the velocity increases over smooth surfaces, with stable running at all velocities up to 5.2 m/s. Zarrouk et al. ICRA 2013.
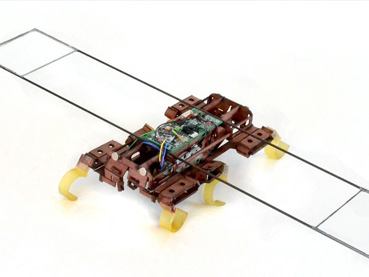
Animal-inspired Design and Aerodynamic Stabilization of a Hexapedal Millirobot
-
(Jan. 2013)
The VelociRoACH is a 10 cm long, 30 gram hexapedal millirobot capable of running at 2.7 m/s, making it the fastest legged robot built to date, relative to scale. Dynamic similarity technique combined with aerodynamic damping provides stability at high speeds. D. Haldane et al. IEEE ICRA 2013.
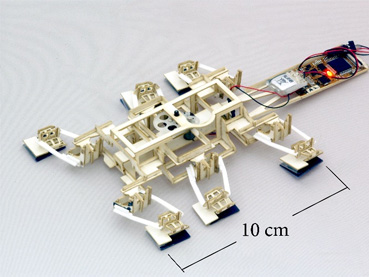
Dynamic Climbing of Smooth Surfaces
-
(Oct. 2012)
Dynamic climbing of near-vertical surfaces introduces reaction forces which require greater normal adhesion to prevent falling. Dynamic climbing on a 30 degree slope succeeds at 12 Hz leg rate, but only 4 Hz on a 70 degree slope. Birkmeyer, Gillies, Fearing IROS Oct 2012
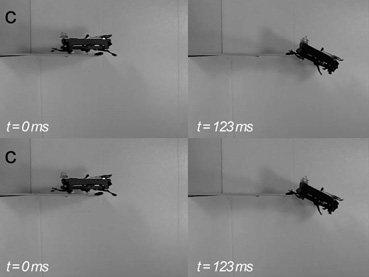
Rapid Inversion: Running Animals and Robots
-
(June 2012)
Cockroaches and geckos can escape when running by swinging under a ledge in a pendulum like motion. The DASH robot, with a special foot attachment, can also swing in a similar manner. Mongeau et. al PLoS June 2012
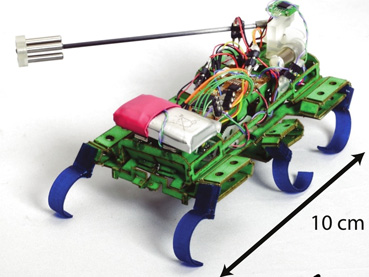
6 legged TAYLRoACH
-
The 100-mm scale Hexapedal robot TAYLRoACH can rapidly maneuver with 90 degrees turns while running. Kohut et al. CLAWAR, Baltimore, July 2012.
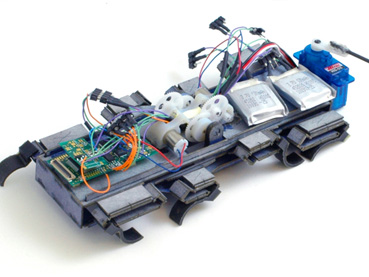
OctoRoACH with Tail
-
The 100-mm scale OctoRoACH robot can turn using differential drive at 100 degrees per second, or with a dynamic tail with peak turn rates of 400 degrees per scond. Pullin et al. ICRA 2012
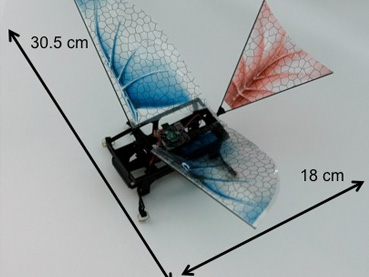
DASH+Wings: Wing Assisted Running
-
(Oct. 2011)
DASH+Wings is a small hexapedal winged robot that uses flapping wings to increase its ground locomotion capabilities. The wings increase climbing slopes and stability, but do not provide enough thrust for flight. A wing assisted running robot and implications for avian flight evolution. Bioinspiration and Bioimetics
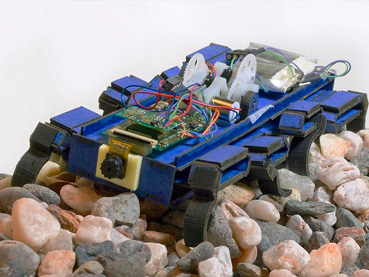
OctoRoACH:Dual Drive MilliRobot
-
(Sep. 2011)
The OctoRoACH robot has a mass of less than 30 grams, and includes the ImageProc CPU with gyro, accelerometer, radio and camera, is capable of locomotion in rough surfaces. Robot designed by A. Pullin. Pullin et al. ICRA 2012
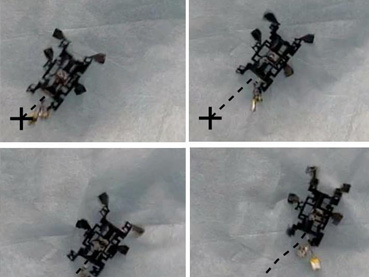
CLASH: Climbing loose vertical cloth
-
(Sep. 2011)
The CLASH robot has a mass of 15 grams, and is capable of climbing a loose cloth surface at 15 cm per second using a simple passive claw mechanism and a coupled in-plane leg drive system. (Birkmeyer et al. IROS 2011)
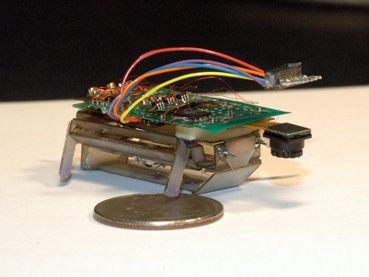
MEDIC Millirobot with body-supported climbing
-
(Dec. 2010)
The Medic robot has a mass of 5.5 grams, and is capable of positioning within 1 mm using static SMA drive. The robot includes camera and wireless.
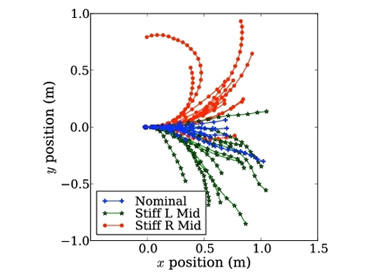
Dynamic turning by modulating leg stiffness
-
(Sep. 2010)
The dynaRoACH robot has mass of 24 grams and is capable of running at 14 body lengths per second. By changing leg stiffness, the robot can execute a 90 degrees turn in 5 leg strides. BioRob 2010
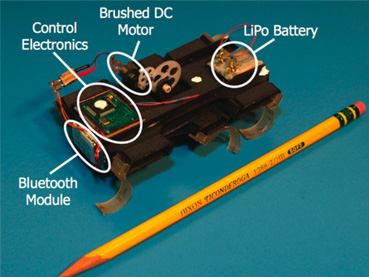
RoACH 2.0 and DASH on Granular Media
-
(Apr. 2010)
Joint work with Goldman lab at GeorgiaTech to measure cost-of-transport on granular media shows 5-30 J/kg-m at 6-10 body lengths per second.
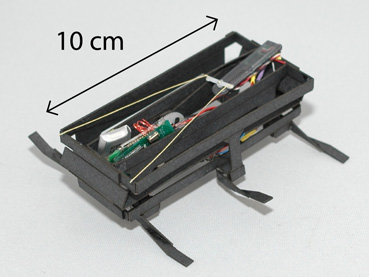
DASH 16 gram Hexapedal Robot
-
(Oct. 2009)
Using compliant fiber board as structural material, and a single main driver motor, the DASH robot is capable of 15 body lengths per second on flat surfaces. The structure is resilient and survives ground impact at terminal velocity of 10 meters per second.
DASH: A Dynamic 15g Hexapedal Robot, IROS 2009.
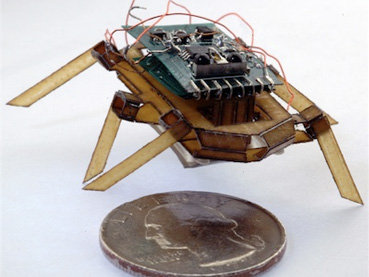
The RoACH Robot
-
In the Biomimetic Millisystems Lab we have combined our expertise in building millirobots with an interest in legged systems to build what we believe is the smallest untethered, legged robot to date - a 2.5 gram legged robot called the Robotic Autonomous Crawling Hexapod (RoACH). This robot makes use of the Smart Composite Microstructures fabrication process and integrated shape memory alloy (SMA) wire actuators. All power, control, and communication electronics are carried onboard and the entire robot is powered with a 20maHr Lithium-polymer battery from the Full River corporation.
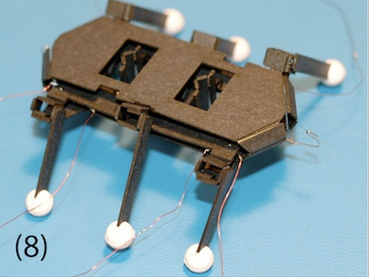
Scaled prototyping of a legged millirobots
-
Using commonly available, inexpensive materials, it's possible to create folded prototypes of legged robots with as many as 60 joints in about 1 hour. The prototypes can then be actuated with DC motors or other types of actuators such as shape memory alloy wire as shown in the photo to the right. Folding Prototyping
Fast scale prototyping for folded millirobots, ICRA 2008
Biologically Inspired Synthetic Gecko Adhesives
-
Micro and nanofiber structures are designed to provide high friction and adhesive forces through mechanical control of surface interactions.
News
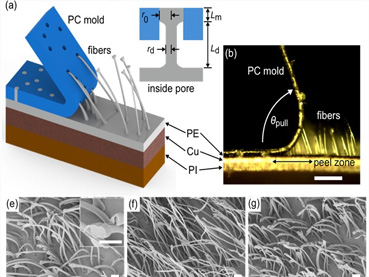
Low-modulus micro fiber arrays
-
(May 2014)
We produced high-aspect-ratio, angled polymer microfiber arrays by directional pulling of polyethylene from low-aspect-ratio, microporous polycarbonate templates. These arrays represent a novel substrate for electrostatic sensors and actuators because they are characterized by low stiffness (<24 kPa effective elastic modulus), low Poisson's ratio (effectively zero at low strains), and very low density (<1% of solid polyethylene).
Angled microfiber arrays as low-modulus, low Poisson's ratio compliant substrates, J. Micromech. Microeng. 24 065016, 2014.
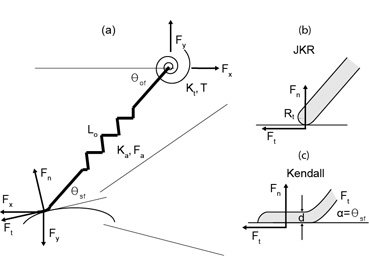
GSA shearing on rough surfaces
-
(April 2014)
A model for the relationship between surface feature size and the adhesive terminal feature shape was developed. On sinusoidal surfaces with amplitudes much larger than the nanoscale features, spatula-shaped features can increase adhesive forces by 2.5 times on smooth surfaces and 10 times on rough surfaces.
Simulation of synthetic gecko arrays shearing on rough surfaces, J. R. Soc. Interface, April 2014.
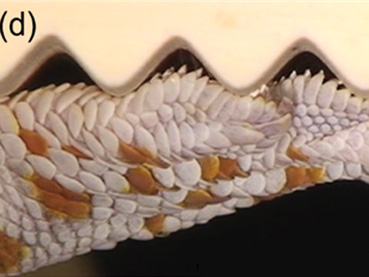
Gecko Toe on Rough Surfaces
-
(Oct. 2013)
We measured the maximum shear force a gecko foot can attain on sinusoidal patterns in sizes similar to the dimensions of the toes and lamellae structures. We found shear adhesion significantly decreased on surfaces that had amplitudes and wavelengths approaching the lamella length and inter-lamella spacing, losing 95% of shear adhesion over the range tested.
Gecko toe and lamellar shear adhesion on macroscopic, engineered rough surfaces, J Exp Biol, October 2013.
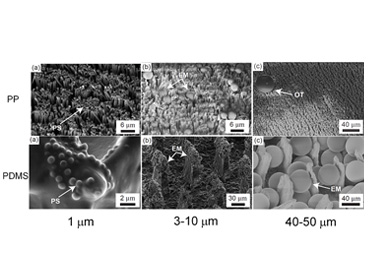
Hard vs Soft Fibrillar Self-cleaning
-
(June 2013)
For certain particle sizes and fiber diameters, both hard (polypropylene) and soft (silicone rubber) fibrillar adhesives can recover adhesive capabilities after fouling with spherical particles. An analysis of the contact strength between fibers, particles and substrates reveals that dry self-cleaning is more effective for smaller fiber diameters and smaller loss functions, such as hard thermoplastics.
Dry Self-Cleaning Properties of Hard and Soft Fibrillar Structures, ACS Applied Materials and Interfaces, June 2013 (just accepted)
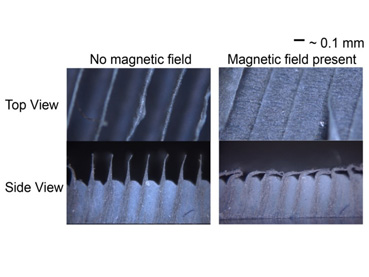
Controllable Particle Adhesion
-
(Feb. 2013)
Controllable adhesion to glass spheres with a magnetically actuated synthetic gecko adhesive is demonstrated. Results show sphere pull-off forces can be increased 10-fold by changing the ridge orientation via the external magnetic field, and that the effective elastic modulus can be changed from 65 kPa to 1.5 MPa.
Controllable particle adhesion with a magnetically actuated synthetic gecko adhesive, Advanced Functional Materials, 2013
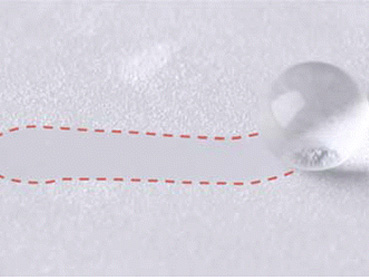
Wet Cleaning of HDPE GSA
-
(Oct. 2012)
Hard-polymer-based GSA formed with high-aspect-ratio microfibers from high-density polyethylene (HDPE) self-cleans with water. The microfiber adhesive shows almost complete wet self-cleaning of dirt particles with water droplets, recovering 98% of the adhesion of the pristine microfiber adhesives.
Wet Self-Cleaning of Superhydrophobic Microfiber Adhesives Formed from High Density Polyethylene, Langmuir, 2012
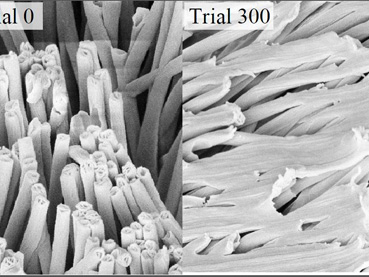
GSA Adhesive Material Limits
-
(Aug. 2011)
HDPE and PP fibrillar arrays have shear adhesion stress (0.3 MPa) sufficient to deform the fibers. Hence the fiber material strength is a limit to greater adhesion strength. Surprisingly, the GSA maintained 54% of original stress in spite or marked deformation over 10,000 cycles.
Shear Adhesion Strength of Thermoplastic Gecko-Inspired Synthetic Adhesive Exceeds Material Limits, Langmuir, 2011
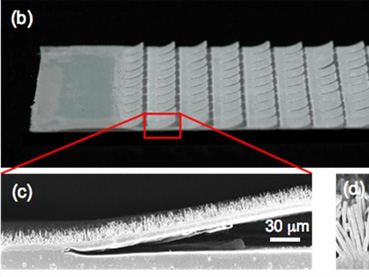
Combined Lamellar Nanofibrillar Array
-
(Oct. 2009)
Lamellar structures act as base support planes for high-aspect ratio HDPE fiber arrays. Nanofiber arrays on lamella can adhere to a smooth grating with 5 times greater shear strength than flat nanofiber array. Langmuir, Oct 2009
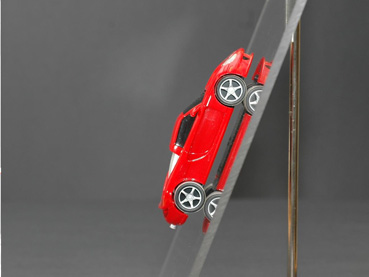
Gecko Tire for Model Car (Nov. 2008)
-
Microfiber array wrapped on model car tire demonstates high friction. (Note: so far, tire only works on smooth surfaces.)
High friction from a stiff polymer using micro-fiber arrays, Phys. Rev. Letters, 2006
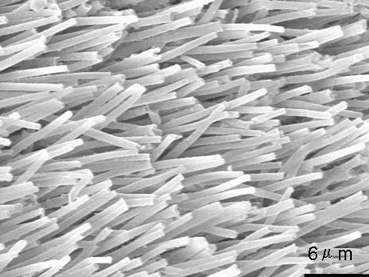
Directional Adhesion of Angled Microfibers
-
(Nov. 2008)
Angled polypropylene microfibers show strong directional adhesion effects, with shear strength in direction of fibers 45 times larger than sliding against fiber directions. A 1 sq. cm. patch supported a load of 450 grams in shear. Directional adhesion of gecko inspired angled microfiber arrays, Applied Physics Letters, 2008.
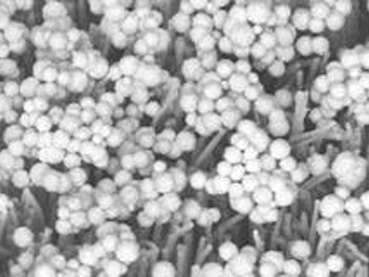
Self Cleaning Gecko Adhesive
-
(Sep. 2008)
First synthetic gecko adhesive which cleans itself during use, as the natural gecko does. After contamination by microspheres, the microfiber array loses all adhesion strength. After repeated contacts with clean glass, the microspheres are shed, and the fibers recover 30% of their original adhesion. The fibers have a non-adhesive default state, which encourages particle removal during contact. Contact Self-Cleaning of Synthetic Gecko Adhesive, Langmuir 2008
Overview of Gecko Adhesion Project
-
Geckos have the remarkable ability to run at any orientation on just about any smooth or rough, wet or dry, clean or dirty surface. The basis for geckos' adhesive properties is in the millions of micron-scale setae on each toe of the gecko form a self-cleaning dry adhesive. The tip of each seta consists of 100 to 1000 spatulae only 100 nanometers in diameter. Our interdisciplinary team of biologists and engineers has been working since 1998 developing models for how the natural nanostructures function in a hierachical combination of spatulae, spatular stalks, setal stalks, setal arrays, and toe mechanics, and developing nanofabrication processes which allow large arrays of hair patches to be economically fabricated.
Synthetic Gecko Nano Hair Properties
-
Using insights from biology, we develop mechanical models for gecko hair adhesion and then design, fabricate, and test micro and nanofibrillar structures. We aim to achieve the seven benchmark functional properties of the gecko adhesive system identified by Autumn (MRS Bulletin 2007):
- anisotropic attachment,
- high pulloff to preload ratio,
- low detachment force,
- material independence / van der Waals adhesion,
- self-cleaning,
- anti-self matting, and
- non-sticky default state.
The low detachment force, self-cleaning, and non-sticky default state suggest hard polymers, rather than the soft polymers typically used in pressure sensitive adhesives. As reported in 2002, [Sitti and Fearing 2002] and Autumn et al. [2002] we have made synthetic spatulae, which have shown adhesion similar to natural spatulae in the range of 100-300 nN. These patches of bumps lacked the setal stalks, and achieved adhesion forces on the order of a few milliNewtons on an area of a square centimeter. In 2003, we fabricated high density arrays of spatular stalks [Campolo et al. 2003] which showed adhesion in shear on the order of 0.5 Newton per sq. cm. In 2006, we demonstrated a novel high friction array of 0.6 micron fibers which showed shear resistance of 4 Newton per sq. cm. with only 0.8 Newton per sq. cm. of normal load [Majidi et al. PRL 2006]. In 2007, we showed how the polypropylene fiber arrays can provide shear force without a normal load being present [Schubert et al. JAST 2007]. In 2008, we made easy-attach easy-release hard polymer gecko adhesives which have a non-adhesive default state [Lee et al. JRSI 2008], and can self-clean during contact [Lee and Fearing Langmuir 2008]. The final goal is to build arrays incorporating the necessary geometrical features which have the same adhesion as geckos to rough and smooth surfaces.
Progress in Gecko-inspired Synthetic Adhesion
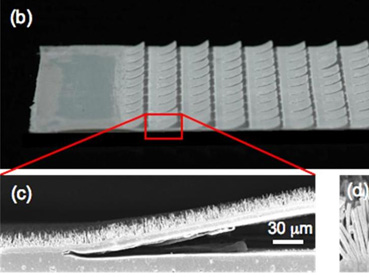
Combined Lamellar Nanofibrillar Array
-
(Oct. 2009)
Lamellar structures act as base support planes for high-aspect ratio HDPE fiber arrays. Nanofiber arrays on lamella can adhere to a smooth grating with 5 times greater shear strength than flat nanofiber array. Langmuir, Oct 2009
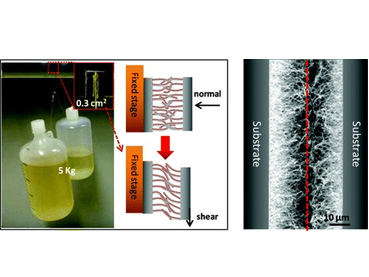
Hybrid CoreShell Nanowire Connectors
-
(April 2009)
Arrays of parylene coated Ge nanowires connect with themselves to form a reusable connector. Uniquely, NW chemical connectors exhibit high macroscopic shear adhesion strength (1.6 MPa) with minimal binding to non-self-similar surfaces, anisotropic adhesion behavior (shear to normal strength ratio 25), low preloading, reusability, and efficient binding for both micro- and macroscale dimensions. Nanoletters, April 2009
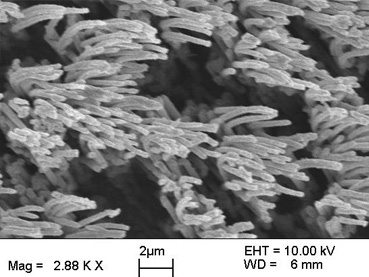
Reduced Resistance Nanowire Contacts
-
(Dec. 2008)
Nickel nanowires can increase the true area of contact and reduce contact resistance. Resistance between a probe and an array of 200 nm by 10 micron nickel nanowires was reduced by an order of magnitude compared to contacting a flat sheet. Reducing contact resistance using compliant nickel nanowire arrays, IEEE Trans. on Comp. and Pack. Tech. 2008.
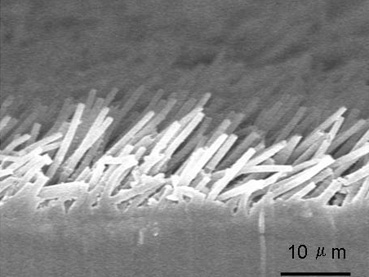
Directional Adhesion of Angled Microfibers
-
(Nov. 2008)
Angled polypropylene microfibers show strong directional adhesion effects, with shear strength in direction of fibers 45 times larger than sliding against fiber directions. Angled fibers also show normal adhesion without shear load, unlike vertical fibers. Directional adhesion of gecko inspired angled microfiber arrays, Applied Physics Letters, 2008.
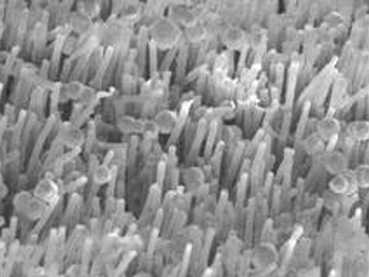
Self Cleaning Gecko Adhesive
-
(Sep. 2008)
First synthetic gecko adhesive which cleans itself during use, as the natural gecko does. After contamination by microspheres, the microfiber array loses all adhesion strength. After repeated contacts with clean glass, the microspheres are shed, and the fibers recover 30% of their original adhesion. Contact Self-Cleaning of Synthetic Gecko Adhesive, Langmuir 2008
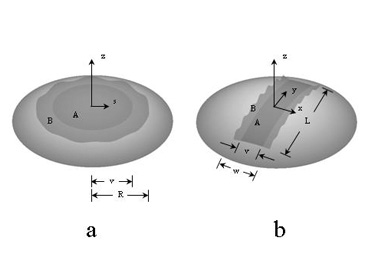
Adhesion of Elastic Plate to a Sphere
-
(Feb. 2008)
Adhesion of even a thin membrane to a non-developable surface, such as a sphere, requires stretching as well as bending. Dividing contacts into small plates reduces membrane strain and increases adhesion.
Adhesion of an elastic plate to a sphere, Proc. Royal Soc. A 2008
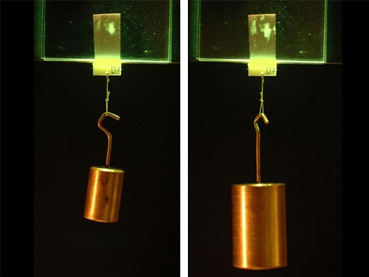
Directional gecko adhesive
-
(Jan. 2008)
First easy attach, easy release, and directional synthetic gecko adhesive using hard polymer microfibers. Microfiber array using 42 million polypropylene microfibers per square centimeter. Patches can support 9 N/sq.cm. in estimated contact region with preload of just 0.1N/sq.cm.
Sliding-induced adhesion of stiff polymer, Interface 2008
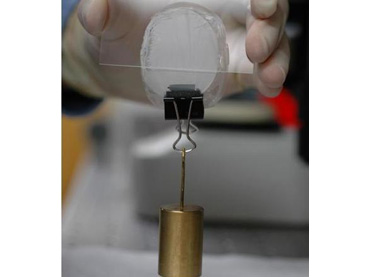
Shear adhesion from polypropylene microfibers
-
(2007)
Shear adhesion of 0.1 N/sq.cm. using 0.6 micron polypropylene fiber array. Normal preload required was less than 0.05 N/sq. cm [Schubert et al. Jnl. of Adhesion Sci. and Tech. 2007].
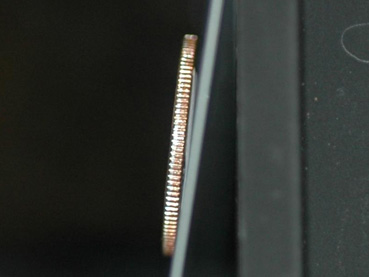
High friction from polypropylene microfibers
-
(2006)
Using an array of vertically oriented polypropylene microfibers, high friction is demonstrated without using a soft/sticky material. At 0.8 N/sq. cm, coefficient of friction is greater than 5.
[Majidi et al PRL 2006 ]. Copyright (2006) by the American Physical Society
High friction from a stiff polymer using micro-fiber arrays, Phys. Rev. Letters, 2006
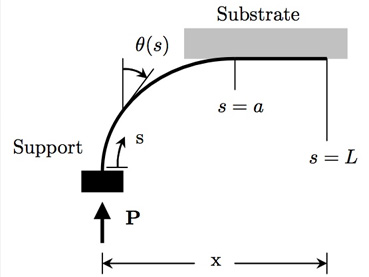
Side contact model for fiber adhesion
-
(2005)
Analysis of fiber adhesion in side contact. [Majidi, Groff, Fearing J. Appl. Phys 2005] shows that sufficiently long fibers, e.g. carbon nanotubes can stably make side contact. This side contact can give 10-20 times greater adhesion force than a hemispherical tip contact.
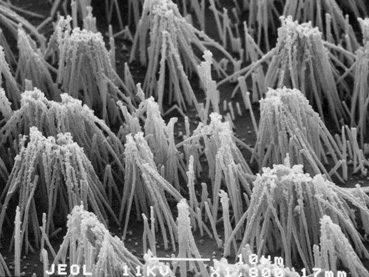
Verification of side-side contact clumping
-
(2004)
Fiber density is limited by clumping- both tip-tip at larger gaps between fibers and side-side for closer fibers. A square lattice is predicted to have better clumping resistance. Polyimide fibers (0.6 micron diameter) showing clumping behavior. [Majidi, Groff, Fearing 2004].
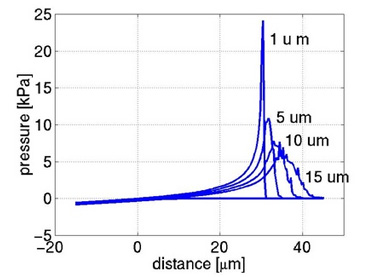
Effect of surface roughness on adhesion strength
-
(2003)
Surface roughness significantly reduces adhesion strength of fibrillar adhesives. A cantilever fiber model predicts a drop in adhesion by a factor of 5 when surface roughness increased from 1 to 15 um.
[Campolo, Jones, Fearing IEEE Nano 2003]
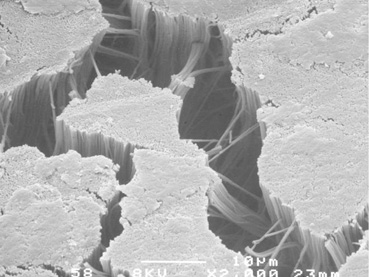
High density nanofibers
-
(2003)
Using a casting process in a template, polyurethane hairs 200 nm diameter and 60 micron long were fabricated. Due to clumping, adhesion force was limited.
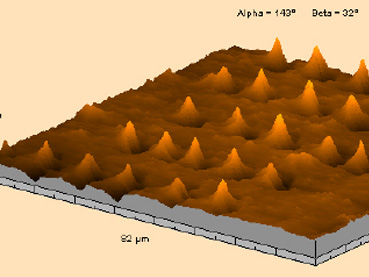
Synthetic spatula array
-
(2002)
Synthetic spatula array from nano-indenting and casting. Approximately 200-300 nN adhesion force was measured per spatula. Total area of array was less than 100x100 sq. um. [Sitti and Fearing IEEE Nano 2002]
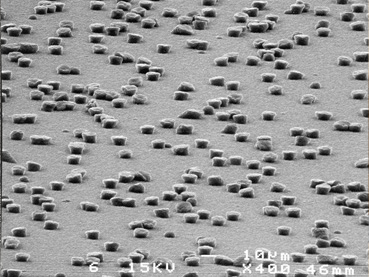
Rubber micro stalk array
-
(2002)
Array of silicone rubber stalks, each 6 um in diameter and 6 um height demonstrated 0.003 N/sq. cm. adhesion and 60 nN adhesion per stalk.[Sitti and Fearing IEEE Nano 2002]
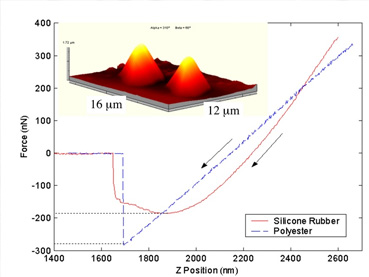
Synthetic spatula
-
(2002)
Single spatula were constructed from silicone rubber (E ~ 0.5 MPa) and polyester (E ~ 1000 MPa) by using nano-indentation and casting. Using atomic force microscope, 290 nN pulloff force was measured from a single polyester spatula with tip radius of 350 nN, and 180 nN for silicone rubber. The similarity of pulloff forces supports the hypothesis of material independence for gecko adhesives. [Autumn et al. PNAS 2002]
Ornithopter Project
-
Flapping flight provides the high maneuverability necessary for operation in a partially structured indoor environment. To achieve robust intelligence for tasks such as search and indoor navigation, the maneuverability of the ornithopter will be combined with a learning approach which makes minimal assumptions about the nature of disturbances and obstacles. This approach will develop optimal control policies for single or multiple vehicles. Based on globally optimal distributed reinforcement learning, we propose to develop algorithms for a set of ornithopters to cooperate in sensing and navigation among unmodelled obstacles such as doors and walls. Our research will be verified with full three dimensional dynamic simulation, a multi-tethered laboratory test-bed, as well as with actual indoor flying ornithopters.
Collaborators:
Prof. Pieter Abbeel, Computer Science Division, UC Berkeley
Prof. Robert Dudley, UC Berkeley
Recent Results
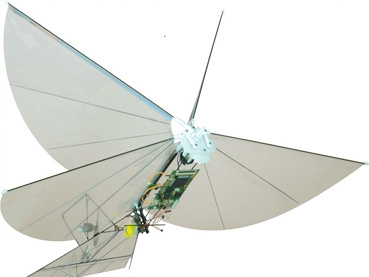
Ornithopter Free Flight Compared to Wind Tunnel Data
-
(June 2014)
We compare the flight of an ornithopter using free flight data collected from a Vicon motion capture system, to measured wind tunnel force and moment values. We determined that the wind tunnel underestimates the angle of attack of the equilibrium point observed in free flight by 15 degrees, while the equilibrium velocity has an error of 0.1 m/s between the two sets at an average flight speed of 2 m/s. (ICRA 2014).
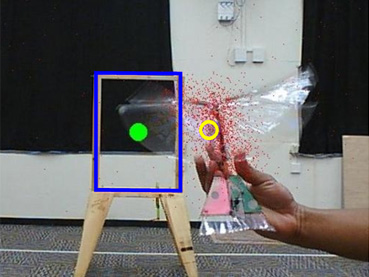
Cooperative Control for Window Traversal with an Ornithopter MAV
-
(Mar. 2013)
We demonstrate cooperative target-seeking between a 13 gram ornithopter, and a lightweight ground station using computer vision. The H2Bird features a carbon fiber airframe, tail rotor, and elevator, and carries a 2.8 gram payload. The ground station provides heading estimates to the ornithopter using a real-time motion tracking algorithm. A model accurately predicts the backwards reachable region for flight through narrow passages. Autonomous Agents and Multiagent Systems (AAMAS2013).
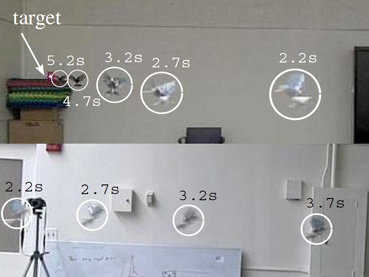
Flight Control for Target Seeking by 13 gram Ornithopter
-
(Sept. 2011)
We demonstrate autonomous flight control of 13 gram ornithopter capable of flying toward a target without any remote assistance. For this demonstration, we have developed a closed-loop attitude regulator for the ornithopter using onboard sensing and computational resources.
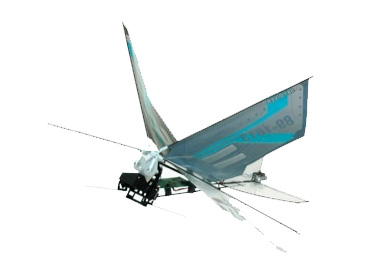
BOLT: Bipedal Ornithopter for Locomotion Transitioning
-
(Sept. 2011)
Bolt is a 13 gram ornithopter with legs for mixed-mode locomotion. In running modes, wings provide passive stability. With wing assisted running, BOLT can run at 2.5 m/sec while maintaining ground contact.
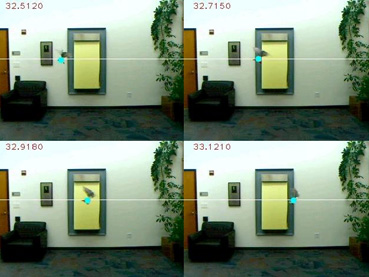
Altitude Regulation of iBird
-
(Sept. 2010)
We identify free flight aerodynamic forces at a stable equilibrium point of an ornithopter and compare them with the tethered flight aerodynamic forces. We developed a closed-loop altitude regulation for the ornithopter using an external camera and onboard electronics. The results show that the tethered aerodynamic force measurement of a 12 gram ornithopter with zero induced velocity underestimates the total flight force by 24.8 mN.
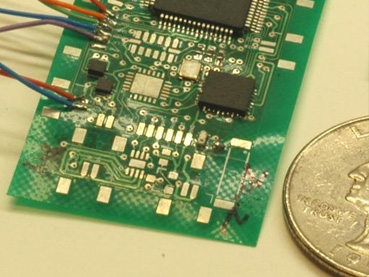
Image Proc 2.2 CPU
-
(August 2010)
Image Proc 2.2 design revision by Stan Baek. Board contains cell phone, gyro, accelerometer, 802.15.4 radio, and 2 channel motor driver in 1.4 grams.
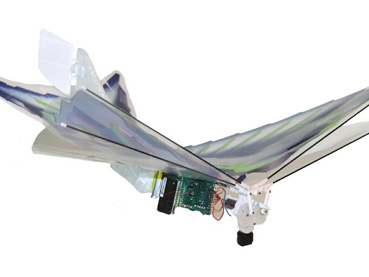
iBird-bot
-
(2010)
Commercially available iBird hover capable ornithopter equipped with ImageProc dsPIC33 CPU board. Total mass 12 grams.
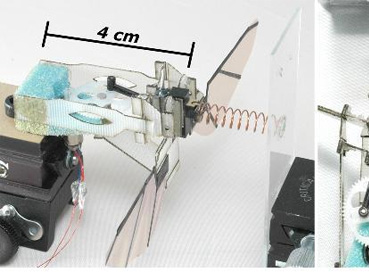
Efficient Resonant Drive of Flapping
-
(Oct. 2009)
A model for a battery-driven DC motor driving a crank is developed, which shows in experiment a 30% reduction in required power when driven in resonance.
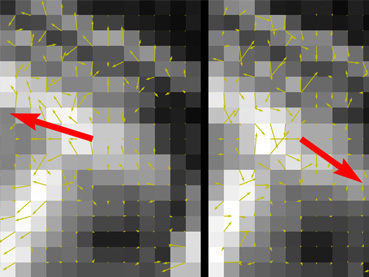
Optical Flow on an Ornithopter
-
(Oct. 2009)
Due to the pitching motion of flapping flight, optical flow has a large superimposed velocity component. This component can be sampled at the wing flapping frequency to recover the underlying signal.
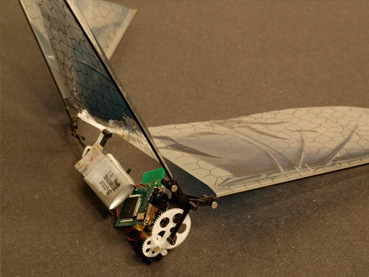
Vamp-bot
-
(2009)
Commercially available VAMP ornithopter with custom low mass electronics. Total mass is approximately 13 grams, including Bluetooth and cell phone camera.
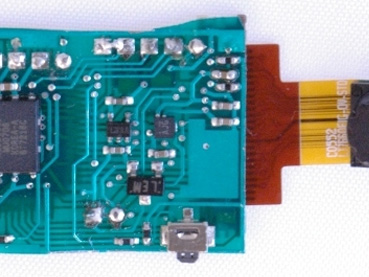
ImageProc 1 CPU
-
(2008)
PIC CPU with Omnivision camera, design by Fernando Garcia Bermudez. Schematic ImageProc v1.0
Folding Prototyping of Meso- and Milli- Robots
-
As the size of a robot decreases, the ratio of its surface area to its volume increases. Because the mass of a robot is proportional to its volume, the increase in this ratio means that surface forces (electrostatic attraction, for example) become large compared to inertial forces. So, as robots (and machines in general) become smaller, friction in their moving parts can become a major source of energy loss, wear, and unpredictable behavior. In the Biomimetic Millisystems lab, we have developed a process called "Smart Composite Microstructures" (SCM) that enables us to build small, strong, lightweight, robots and structures whose ability to move comes from bending of compliant polymer hinges that connect rigid links made from carbon fiber and other composites. These structures are made as single flat pieces and are folded up to form more complicated shapes and linkages. They can also be integrated with smart actuators like piezoelectrics and shape memory alloy to provide motion.
Details on the SCM process can be found in: R.J. Wood, S. Avadhanula, R. Sahai, E. Steltz, R.S. Fearing
ASME Journal of Mechanical Design, vol. 130, no. 5, 2008. [link]
For cardboard: SCM: A.M. Hoover and R.S. Fearing, A Fast Scale Prototyping Process for Folded Millirobots IEEE Int. Conf. Robotics and Automation Pasadena, May 2008. [link]
Prototyping Folded Robots
-
Even with the SCM process, very small robots can be difficult to design and build. Their size makes assembly challenging and the inherent difficulty of designing a 3 dimensional folded robot in a 2 dimensional drawing also slows the process. To avoid costly errors in the early stages of design when many ideas will be tested and discarded, we created a scaled analog to the SCM process using commonly available materials. This scaled process lets the folded robot designer go from a design on paper to a functional scaled prototype in as little as 20 minutes. Rapid iteration alleviates the risk of committing to a design and fabricating at the small scale too soon. Instead, the designer is free to explore a variety of ideas at the larger scale, discarding the unsuccessful attempts and rapidly integrating lessons learned in the process to produce a design that is much more likely to succeed at the small scale.
The Prototyping Process: Step by Step Illustration with Hexapod Crawler Example
-
Required Equipment and Supplies
2D CAD software (Solidworks, CorelDraw, etc)
VersaLaser laser cutter
Posterboard
Glue stick/hot mount adhesive
Polyester film (0.001" - 0.004" thick)
Glue (white, or cyanoacrylate) 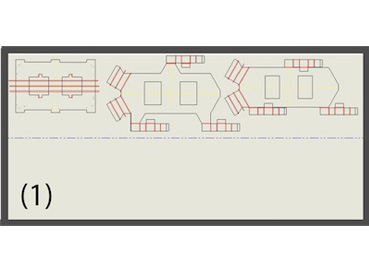
Step 1: The Drawing
-
The process begins with a 2 dimensional drawing in a program that supports vector graphics. In the lab we use the 2D CAD program, Solidworks. It is also possible to use a program like Corel Draw. However, Solidworks is preferred because it gives explicit control over dimensions and allows the user to define relations between entities within the drawing. Lines that will become flexure hinges in the robot are colored red while lines representing the outlines of the part are colored black. Blue lines are for squaring and scoring the workpiece. These lines are cut at different times - the reason for this is explained in the following steps
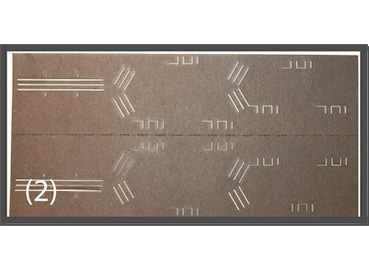
Step 2: Cutting the Flexures
-
The blue lines are first cut out to square the workpiece and create a fold line in the middle. The workpiece is folded and the flexure cuts are made, creating mirrored cuts in the workpiece shown in the picture to the right.
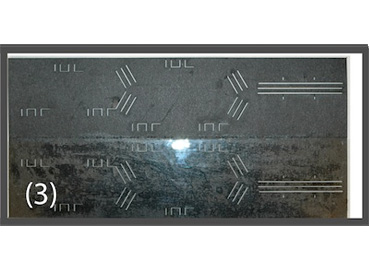
Step 3: Flexure Layer Insertion
-
Glue is spread over the inner faces of the workpiece and a piece of polyester large enough to cover all flexure cuts is placed over one of the inner faces. (Alternatively, the posterboard can be prelaminated with hot mount film on the inner faces.) The workpiece is folded up, sandwiching the polyester film between the two sides. Care should be taken to align the flexure cuts. Alignment can be checked by holding the folded piece up to a light
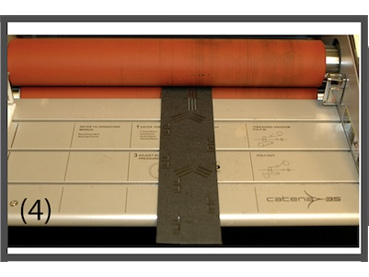
Step 4: Laminating
-
The folded workpiece is passed through a hot laminator with maximum pressure exerted by the rollers. This step ensures even bonding of the posterboard to the polymer flexure film. The resulting sandwich is now ready to have the part outlines cut on the laser cutter.
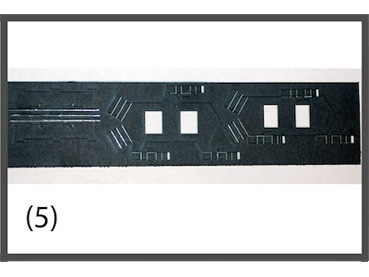
Step 5: Cutting the Outlines
-
The sandwich is placed back in the laser cutter. It is important to place the workpiece back in the same orientation as in Step 2 when the flexures were cut. The outlines of the parts are cut now. The picture to the left shows the parts with their outlines cut, but not removed from the workpiece.
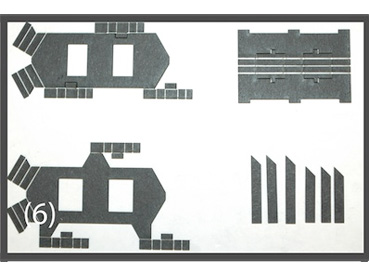
Step 6: Releasing the Parts
-
The parts can now be popped out of the workpiece. The results are integrated, articulated parts with hinges where the flexure cut lines were placed in the drawing and rigid posterboard links between.
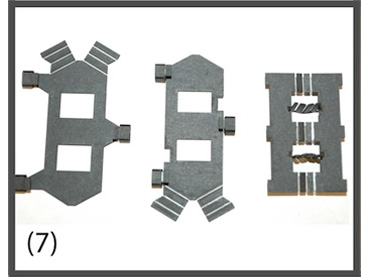
Step 7: Pre-folding Linkages
-
If any of the parts contain linkages that can be folded before the parts are joined, they can be folded and glued at this point. In the picture on the left, fourbar linkages have been created by folding up the links attached to the two parts on the left. These fourbars will form the hips of a six-legged robot when the entire structure is assembled. The part on the right has been folded into a Sarrus linkage. This linkage sits in the middle of the finished robot and by contracting and expanding serves to lift and lower the two sets of three legs (tripods).
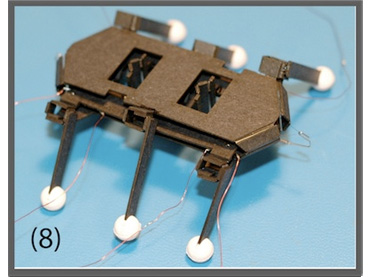
Step 8: Final Assembly
-
Individual parts or subassemblies can now be assembled. In the photo on the right, the three plates from Step 7 are glued together. The plate on the far left is on the bottom, the Sarrus linkage is in the middle, and the plate in the middle of the picture in Step 7 is on top. Legs have also be glued to the fourbar hips and the ends of the legs have been fitted with spherical silicone rubber feet.
Example Structures
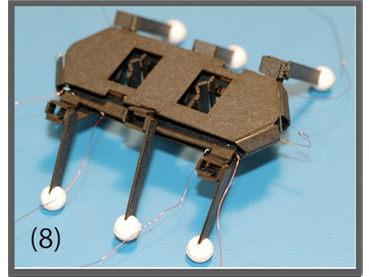
SMA Driven Crawler
-
These are the files used to cut out the shape memory alloy driven crawler shown in the movie above.
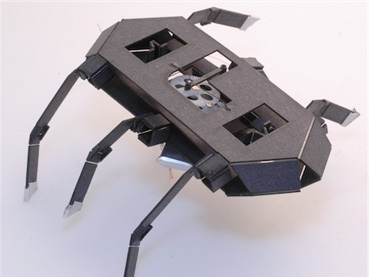
Motor-Driven Hexapod
-
A DC motor can also be integrated with the crawler assembled in the step-by-step instructions. This crawler uses the same kinematics, but is modified to accommodate the motor and gear train. Flexure hinges can also be used as springs. In this prototype, a slightly bent knee is added to the leg to enable a small amount of compliance.
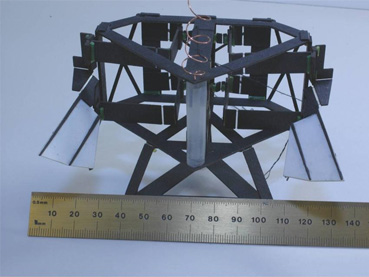
5X Scale Mockup of Micromechanical Flying Insect
-
The micromechanical flying insect uses two actuators per wing. Each actuator drives a slider crank connected to a 4 bar. A pair of 4 bars drives a spherical 4 bar which acts as the wing hinge. Each wing has 2 DOF and uses a 15 joint linkage. In total, the mockup uses 30 flexure joints, and another 30 fixed joints for the structure/air frame.
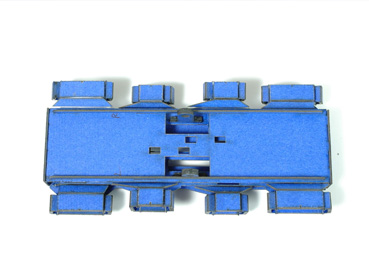
OctoRoACH robot
-
The OctoRoACH robot design by A. Pullin (Pullin et al. ICRA 2013). OctoRoACH uses 1 DC motor per side. Transmissions can be found in small flying toys such as VAMP.
Prototyping using other Materials
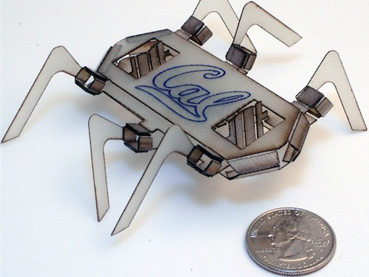
...
-
While posterboard is a convenient material that is readily available, inexpensive, and reasonably strong, for actuated models, a more robust engineering material is preferable. We have recently extended this process to use G10 fiberglass. the G10 provides a higher specific modulus than cardboard and is more robust when subjected to repeated actuation cycles. The use of fiberglass also opens the possibility of integrating printed circuit boards directly into the robot's skeleton.
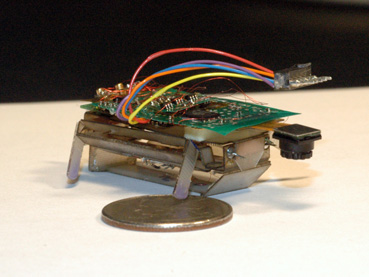
MEDIC Millirobot with body-supported climbing
-
(Dec. 2010)
The Medic robot is fabricated from thin fiberglass sheets, and has a mass of 5.5 grams, and is capable of positioning within 1 mm using static SMA drive. The robot includes camera and wireless.