Italian Institute of Technology (IIT)
Videos
Loading the player ...
- Offer Profile
- Welcome to Robotics, Brain
and Cognitive Sciences
The research activity of RBCS develops around “the human being” along three
main research streams: humanoid robotics with a focus on cognition, human
behavioral studies and rehabilitation with a focus on action and perception,
human-machine communication and interaction with a strong emphasis on the
technological and scientific advancement of bidirectional direct interface
to the nervous systems. RBCS is the house of iCub
Product Portfolio
Robotics, Brain and Cognitive Sciences
Labs
- Within this framework RBCS is organized in a number of
laboratories addressing, each with its own specificities and investigation
tools, topics like: learning and adaptation, multimodal perception and
affordance, motor control and learning, natural and social interaction,
attention and recognition. The three main streams of research are mapped
into the following laboratories:
Study Humans: Human Behavior and Rehabilitation Labs
Research in this stream will focus on understanding aspects of human
behavior and perception which, besides their scientific value, are essential
for the implementation of complex behaviors in artificial systems and new
motor rehabilitation devices. All the research aspects addressed have in
common the goal of understanding not only the motor and/or perceptual
“process” per as but also how the processes adapt, learn and develop as a
consequence of interaction with the environment and other human beings.
Build Humanoids: Cognitive Humanoids Lab
The main focus of the Cognitive Humanoids Laboratory activities is in the
implementation of models of cognition in robots of humanoid shape. This has
the two-fold aim of i) furthering our understanding of brain functions and
ii) realizing robot controllers that can learn and adapt from their
mistakes.
Interaction and Interface: Interaction and Interface Labs
The main effort in this area will be devoted to the study of chronically
implantable Brain Machine Communication devices in humans. In particular the
main focus of this long term goal is to implement bidirectional and “ad-hoc”
interfaces. By this we mean interfaces that can be adapted to the residual
functional abilities and the morphology of individual patients (up to the
shape of the “connector”) and that can support bidirectional flow of
information between the nervous system and the artificial device.
Cognitive Humanoids Lab
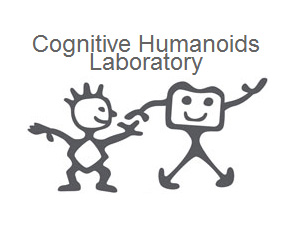
- Scientists at the Cognitive Humanoids Laboratory work
at the forefront of the robotics and neuroscience research implementing
models of cognition in robots of humanoid shape. This heterogeneous group of
people aims at understanding brain functions and realizing robot controllers
that can learn and adapt from their mistakes.
Activities encompass the construction of the hardware, that we call "bodyware",
and software which will make, one day, machines of intelligence comparable
to humans. We call this technology "mindware".
On the bodyware side we developed the iCub, a humanoid robot, shaped as a 4
years old child. Simultaneously, we are addressing the development of the
technologies for the next generation of robots based on soft and adaptable
materials both for sensing and processing.
On the mindware side, the laboratory is involved in the realization of the
cognitive skills of the humanoid robot; that is, providing the robot with
visual, auditory and tactile perception and the ability to gaze, reach and
manipulate objects.
Bodyware: the iCub
- The iCub is the humanoid robot developed as part of
the EU project RobotCub and subsequently adopted by the Cognitive Humanoids
Laboratory at IIT. It has 53 motors that move the head, arm & hands, waist,
and legs. It can see and hear, it has the sense of proprioception (body
configuration) and movement (using accelerometers and gyroscopes). We are
working to improve on this in order to give the iCub the sense of touch and
to grade how much force it exerts on the environment. Simultaneously, we are
already planning the next generation of humanoid robots: they will be made
of soft actuators, new stronger and lighter joints with power efficiency
closer to that of biological systems like ourselves.
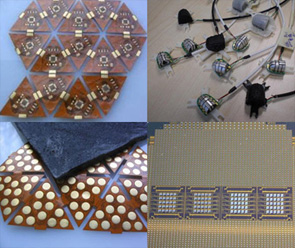
Skin
- Tactile sensing is one of the key missing technologies
for humanoid robots to interact safely in everyday environments. We are
investigating various technologies with the aim of constructing a
full-fledged robotic skin that includes seamless integration of sensing,
wiring and processing. The realization of a robotic skin is made complicated
by the necessity of covering a wide area, conforming to the shape of the
body while standing considerable mechanical stresses (including impacts).
Data have to be collected from this distributed network of sensors and made
available at various levels of processing for reactive control and planning.
Further, tactile data have to be combined with other modalities like
proprioception and force/torque measurement. Our current solutions are based
on capacitive and piezoelectric sensing both at the millimeter and
micrometer scale (VLSI).
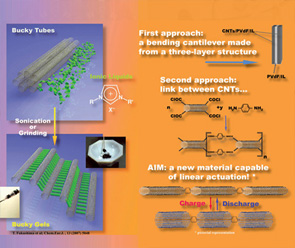
Materials
- From nano actuation to macro actuator
Carbon Nanotube actuators - The main issue in developing devices based on
nano scale actuation mechanism is the transfer of stress induced at the
level of the single Carbon Nanotube (CNT) to the macroscopic domain. A
possible approach to this issue is to. The transfer of stress induced at the
level of the single CNT to the macroscopic domain can be achieved by a novel
approach to the problem. Instead of simply using van der Waals interaction
between CNTs or merely incorporating in a polymeric matrix, the idea is to
link CNTs via chemical bonds in order to create a copolymer with single
nanotubes as repeating unit. To this end one possible route is to create two
different kinds of CNTs, one functionalized with carboxylic group and the
other amino substitutes; these moieties can react without any addition of
catalyst as in the synthesis of polyamides (i.e. Nylon 6,6). An easiest
route to link CNTs via chemical bond is to use a linker molecule, i.e. an
aromatic diamine, that could react with acyl chloride (-COCl) modified CNTs
as shown in the above scheme.
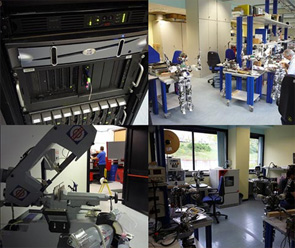
Infrastructure
- The Cognitive Humanoids Laboratory relies also on
mechanical, electronic and informatics support both internally and through
the shared resources of the RBCS department. In particular, our technical
infrastructure helps in developing new mechanical and electronic components,
debugging existing solutions, and to maintain our robots and computational
infrastructure. Mechanical development is carried out together with the
mechanical workshop facility, electronics is developed in collaboration with
the electronics design laboratory and the computer network is maintained
together with the RBCS system administration group. We also developed the
software and firmware infrastructure of the iCub (see for example yarp).
The current design focus on the development of a new release of the iCub
called tentatively “v2” which includes force/torque control, a skin, and
several mechanical improvements including bipedal walking capabilities
(together with the advanced robotics department).
Mindware: the technology of cognitive systems
- Our real interest is of course in the eventual
realization of intelligent machines. We research on the basic technology of
cognitive systems and on its translation into a full working system. Our
modus operandi in this case starts from biology, that is by "reverse
engineering" the brain, continues through mathematical modeling of specific
brain functions that finally find an implementation on the humanoid robot.
Examples of the cognitive skills that we study are attention, reaching and
manipulation. Although broad in scope this research has a clear and
measurable target also of industrial interest. In particular, humanoid
robots can be imagined as helpers in manufacturing, office or home
environments. In this respect, for example, we address safety in
human-machine interaction (at the cognitive, control, and hardware level).
We also participate to projects that will add language skills to the ICub
and we are developing microelectronics for sensing, actuation and
processing.
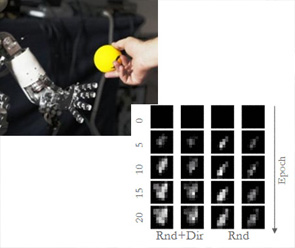
Development
- Our stance on cognition coincides with the emergent
systems approach: cognition is the process whereby an autonomous system
becomes viable and effective in its environment. From the implementation
point of view, we see cognition as the ability to predict the future
unfolding of events initially dealing with the immediate (closer to direct
sensory perception) and gradually developing to span a longer time frame
(where sensory perception alone cannot get). Cognition cannot be hand-coded,
it is necessarily the product of a process of embodied development. Thus,
studying and modeling learning and development is a crucial aspect of our
research agenda.
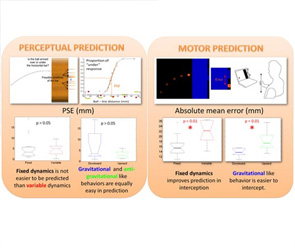
Motor control
- Motor control has a predominant role in the understanding
of cognition [ref 1]. We employ various methods ranging from machine
learning and non-linear optimization to investigation of human behavior (in
collaboration with the human behavior lab). On the computational side, this
is done using parametric and non-parametric techniques. The human behavior
aspects are covered relying on the equipment of the human behavior lab for
measuring kinematics of the arms and body, eye movements, and
electromyography.
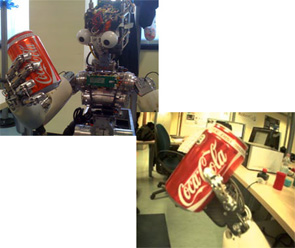
Affordances
- Vision and manipulation are inextricably intertwined in
the primate brain. Tantalizing results from neuroscience are shedding light
on the mixed motor and sensory representations used by the brain during
reaching, grasping, and object recognition. We now know a great deal about
what happens in the brain during these activities, but not necessarily why.
Is the integration we see functionally important, or just a reflection of
evolution’s lack of enthusiasm for sharp modularity? We instantiate these
results in robotic form to probe the technical advantages and to find any
lacunae in existing models. We believe it would be missing the point to
investigate this on a platform where dexterous manipulation and
sophisticated machine vision are already implemented in their mature form,
and instead follow a developmental approach from simpler primitives.
Visuo-haptic Perception Lab
- All senses provide simultaneous information about our
environment and this information needs to be combined into a single percept.
The focus of the activity of the lab is the study of unimodal visual,
tactile and auditory perception in humans their multimodal integration. We
principally investigate the integration between visual and tactile signals
with the goal of understanding the rules that govern the merging of
perceptual information mediated by the intention to move signals and prior
knowledge. Such aspects are relevant for the understanding of human
perception as well as for the implementation of artificial systems.
Furthermore the interest of our research is extended at the analysis of the
dynamic of these perceptual capacities during development in normally sight
children and considering patients with visual disabilities. 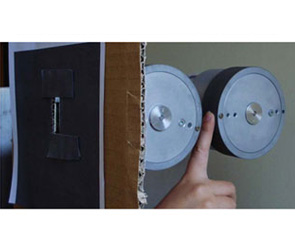
Figure 1: haptic devices designed at human behavioiur lab at IIT.
- A-Braccio di Ferro BDF in bimanual configuration, B-Haptic Grasp unit, C- IIT Wrist Robot, D- 12DOFs Haptic Workstation for Bimanual manipulation of distal and proximal arms.
Motor Learning and Rehabilitation Lab
- The activity of the Lab is focused on the study of the
neural plasticity that underlies the organization of the human sensorimotor
system and its capacity to learn motor skills in the context of a complex
environment. This also includes cognitive aspects in the neural control of
movements because we believe in “embodied intelligence”: we see adaptive
behavior as the emergent property of the bi-directional interactions of the
nervous system with the body and the environment. This implies a continuous
exchange of signals/energy between the nervous system, the body and the
environment and it means that the motor neuronal input is shaped by the
biophysics of the sensory organs and the motor neuronal output is
transformed by the biomechanics of the body creating, at the same time, a
large set of constraints & affordances.
Within this background the Lab investigates paradigms of motor learning and
control by using visuo-haptic, dynamic virtual environments and measuring
the motor response (in terms of kinematics and kinetics) and the
corresponding neural correlates (in terms of muscle activity –EMG- and brain
activity – EEG and NIRS).
The haptic part of the virtual reality system is provided by a several
haptic robots (figure1) that have been designed in the lab (BdF in mono- or
bi-manual configuration, IIT-Wrist robot, haptic grasp unit).
Inter-limb interference during bimanual adaptation to
dynamic environments
- The two robots generate two independent curl viscous
force fields that tend to deviate the hands laterally in the clockwise or
counterclockwise directions. The focus of the study is on the patterns of
interference between the two learning processes (for the left and right
hands, respectively).
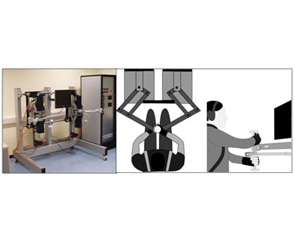
Figure 2 Experimental set-up
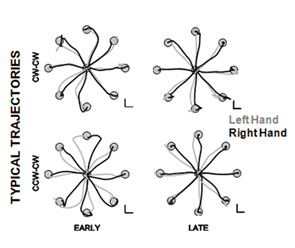
Figure 3: Movement trajectories at the beginning (left) and end (right) of the force field adaptation phase, for two typical subjects in the CW-CW group and CCW-CW group. Lines in grey and black denote, respectively, the left and right hand. Scale bar: 2 cm.
Visuo-proprioceptive integration for compensating
dynamic perturbations of wrist pointing movements
- The subjects perform pointing movements with their
wrist, with various combinations of visual/kinesthetic perturbations input
by means of the visual feedback and the wrist device.
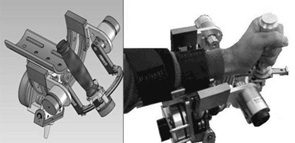
Figure 4: IIT Wrist Robot.
- It has 3 DoFs: Flexion/Extension, Pronation/Supination,
Adduction/Abduction. One motor is used for F/E and P/S; two motors for A/A.
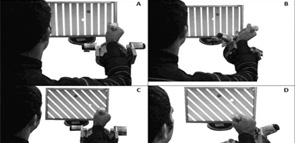
Figure 5: Wrist robot and Virtual Reality.
- Visual representation of the target position and the
actual pointing direction, as two circles, in relation with the manipulandum
degrees of freedom. The two circles are visualized against a structured
background (a stripe pattern). The overall picture could be fixed, with
respect to the computer screen, or rotated in an harmonic manner. A:
familiarization setup, B:kinesthetic disturbance; C: visual disturbance; D:
visuo-kinesthetic disturbance.
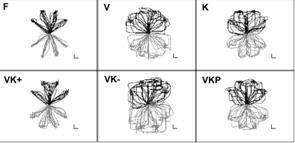
Figure 6 example of trajectories for different target
sets.
- Black trajectories start from the center to each
peripheral targets while grey trajectory are towards the center from the
peripheral four targets and are mirrored below in the figure for graphical
clarity sake; in (A) Familiarization (F), (B) visual (V), (C) kinesthetic
(K) , (D) visuokinaesthetic (VK+), (E) visuo-kinaesthetic 180° phase lag (VK-)
and (F) visuo-kinaesthetic 90° phase (VKP); target set (scale bar 2.5 cm).
Minimally assistive reaching strategy in robot therapy
- The robot helps severe hemiplegic patients to perform reaching movements by generating assistive force fields of minimal intensity, in order to foster the emergence of active, goal oriented movements in a quasi-paralyzed limb.
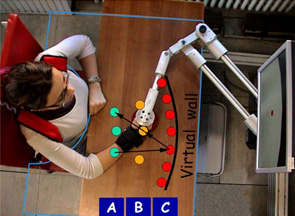
Figure 7 A view from above of a subject holding the
manipulandum.
- The subject’s shoulders are strapped to a chair; the
forearm is attached to a sliding support; the wrist is stabilized by means
of a skateboard wrist brace and the hand grasp by means of a Velcro holder.
The targets are arranged on three layers: A, B, C. The C layer is placed in
front of a virtual wall.
The basic sequence of target activation is A - C - B - A and it is repeated
3 9 7 9 3 = 63 times in a random order.
Note that the target distance in the figure is twice the real distance for
graphical reaso.
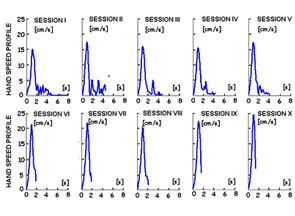
Figure 8 Evolution of the speed profile from the initial
to the final session, performed by a subject for the same movement (from the
central position of the A layer to the central position of the C layer).
- In general, all the subjects were characterized by a
trend to quicker and smoother reaching movements in spite of the fact that
the level of the assistance force was progressively reduced
Adaptive robot assistance in tracking movements
- The robot helps the patient in tracking a moving target (simple Lissajous figure-of-eight) with an assistive force field that is modulated automatically as a function of the performance.
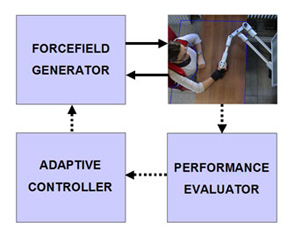
Figure 9: The Force field generator uses an impedance
control scheme, with the direct drive of the robot actuators, in such a way
to transmit to the handle a force vector computed as a function of the
kinematic state of the robot (sampling frequency: 1 kHz).
- The Adaptive Controller modulates the gain of the force
field as a function of the evaluated performance, according to a
non-monotonic training protocol. Continuous vectors: continuous time
control; Dotted vectors: intermittent control.
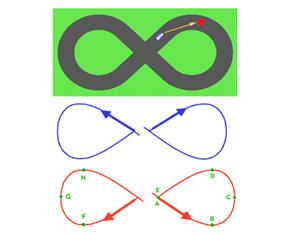
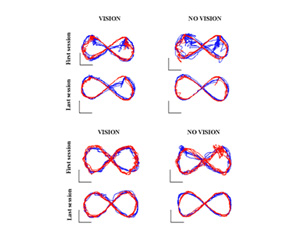
Figure 10 Left: The top panel replicates the picture on
the computer screen that includes the figure-of-eight path (black), the
moving target (red circle), and the hand position (whitish car-shaped). The
middle and bottom panels show the two tracking directions used in the
experiments:
- CWright_CCWleft (blue), CCWrightCWleft (red). A – H are
the eight control points used by the algorithm of performance evaluation.
Right - The top set of 4 figures is related to a subject with a sever
impairment level (FMA= 4). The bottom set of figures is related to a subject
who is affected in a lighter way (FMA= 25). Blue denotes the
clockwise-right/counterclockwise-left sequence; Red denotes the
counterclockwise-right/clockwise-left sequence.
Action and Perception Lab
- The main focus of the scientific activities of the lab
is to advance the understanding of the mechanisms involved in the production
of goal oriented actions. The working hypothesis is that action and
perception processes are strongly linked. Consequently action production is
studied by considering both motor command and perceptual feedback.
Perception is investigated through several experimental paradigms based on
the idea of online mental simulation of the observed action. Action is
studied by considering simple (arm) and whole body movements, where
empirical observations are associated to numerical simulations. Among
several specifics questions that are addressed we try to understand how the
gravity force field is represented at the different levels of the CNS. A
potential cognitive representation is studied using the recorded kinematic
and muscle activity of simple arm reach to grasp movement performed in a
real or a virtual environment. A sensorimotor representation is also
considered by studying the multijoint body mechanical system during a whole
body reach to grasp motion. The existence and the combination of specific
motor primitives is investigated in the frame of our understanding of the
coordination of reaching and equilibrium subgoals inherent to this complex
task. The plasticity of action production is investigated after hypo
activity and learning. Functional (motor efficiency through kinematic
analysis) and structural (brain plasticity through transcranial magnetic
stimulation SEP and MEP recording) experiments are conducted simultaneously.
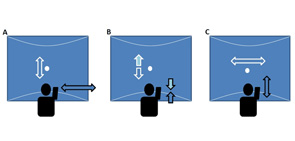
Effect of visual context on arm motion planning
- It is well known that visual information is necessary to
execute accurate goal-directed tasks. Other sensory inputs as proprioception
and vestibular information contribute as well, elaborating the initial state
of the sensorimotor system during motor planning and keeping the on-line
control of execution. The relative roles of vision and proprioceptive
inputs, however, have still to be quantified. To this aim we investigate the
influence of visual feedback on action planning by means of a visuo - motor
conflict.
Visuo motor conflicts presented in the experiment: A) Horizontal arm motion
(black arrow) with vertical visual feedback (white arrow) B) Vertical arm
motion (black arrow) with vertical visual feedback in opposite direction
(white arrow) C) Vertical arm motion with horizontal visual feedback (white
arrow) .
We ask subjects to perform vertical and horizontal single-joint arm pointing
movements, while they visually perceive either congruently or incongruently
(e.g. orthogonally) directed movements. The changes in the arm velocity
profiles produced by this visual feedback manipulation represent a good
measure of the effect of a conflicting visual information on motion. This
will allow us to check whether visual information can reorganize motor
planning and change motion kinematics.
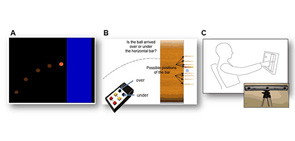
Perceptual and motor bases of prediction
- Prediction is a central skill in human life. As our
environment is constantly changing, both as a consequence of our actions and
independently of us, it’s necessary to anticipate when and where future
events will happen, in order to be able to synchronize our actions with them
and to proficiently interact with the world. The study of interception
abilities represents a good option to investigate this topic, as
interception is a quite common task and, at the same time, it strongly
requires anticipation skills.
Methods and apparatus of the experiments on prediction: A) The visual
stimulus, i.e. a virtual target moving on a screen. B) Purely visual task
schema and response box used by subjects to answer. C) Interception task
schema and motion tracking cameras (Optotrak system) used to record index
finger motion.
In our experiments a ball disappears behind an occlusion after following
parabolic paths. Subjects should either select where the ball would arrive
after the occlusion (only vision involved) or directly intercept the virtual
target by pointing on it with their index finger (vision plus motor
involvement). This way it is possible to understand whether the goal of a
predictive effort affects how prediction is performed and if an internal
model of target motion is built and exploited in prediction.
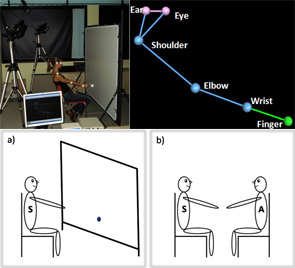
Action and perception coupling
- The general aim of this research theme is to understand
the organizational principles sub serving the control of natural
goal-oriented actions and how this control is implemented at physiological
level. Two different experimental paradigms are applied: biological motion
recognition and motion imitation.
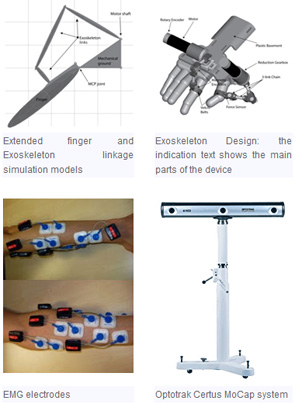
Dexterous control of a robotic hand
- The stiffness - the change in resistive force that
counteracts the effect of external loads - is substantially dependent on
variability in limb geometry and orientation, and on antagonist muscles
co-contraction. The control of joint impedance provides mechanical stability
in presence of external perturbations and this is the reason why active
modulation of limb impedance by the central nervous system (CNS) is
considered an essential part of effective motor control.
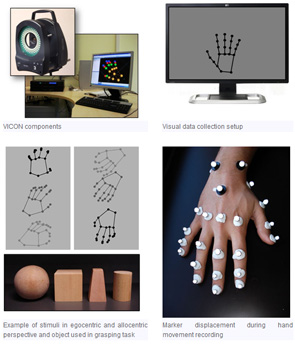
Object feature extraction
- In our everyday life we reach, grasp and manipulate many
different objects, and we also look at other people executing these same
action. The relationship between some intrinsic object features and the
temporal coordination of joints` motion during reach to grasp tasks are
extensively described in literature by a growing corpus of researches. Human
sensibility to the biological motion is also extensively studied, with a
focus on the capability to recognize human actions and their subtle details
from highly impoverished stimuli, like ‘point-light displays’.
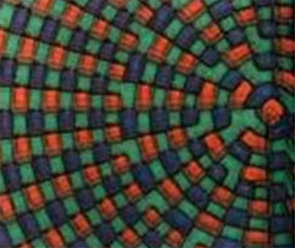
Cortical and functional plasticit
- Michela here is working to better understand if a brief
period of hand non-use may induce modifications in the motor performance
during a grasping task and if cortical plastic changes occur in the brain.
To this issue, we immobilized subjects’ hand with a soft bandage used in
everyday clinical practice by physiotherapists.
Brain Machine Interface Lab
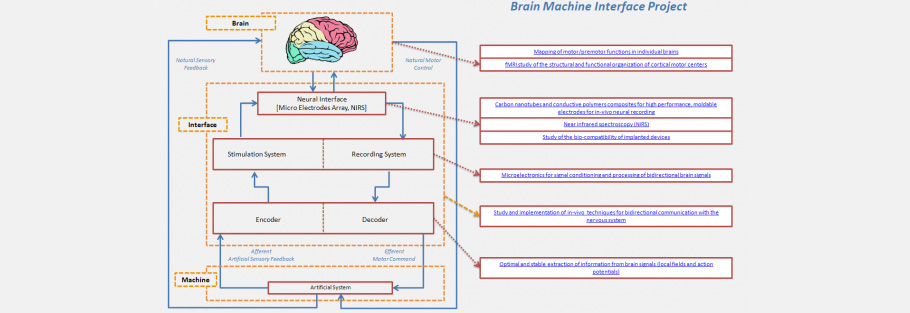
- This laboratory sets out to identify research paths
that can effectively lead to the development of an artificial system capable
of interacting with the ambient under cerebral control. Its distinctive
characteristic lies in the fact that it integrates different approaches,
making it particularly suitable for a research environment such as that of
the Italian Institute of Technology (IIT). A peculiar feature of the
proposed approach is the acquisition of in vivo data. This is because,
though the project is considered of as a source of fundamental data for
basic research, it is and remains an applicative project incorporating major
technological challenges. As all the stages of the research project carried
ou in the BMI lab are applicable to the man, this predetermines the choices
of the materials and procedures required for its realisation. Although the
majority of the research is performed within the Department of Robotics,
Brain and Cognitive Science, the project benefits also from the contribution
and collaboration of various other branches of the IIT, such as Neuroscience
and Nanotechnology. Specifically, as regards those project skills that
cannot be found within the IIT, use has been made of a network of
collaborators including some leading Italian research centres. Among them,
the Politecnico of Milan Electronics (POLIMI-ele) and Physics (POLIMI-phys)
Departments, the Neurophysiology Sections of the Universities of Ferrara (UNIFE)
and Modena (UNIMO), the Neurosurgery Department of the Udine Hospital “S.M.
della Misericordia” (NSG), the SISSA in Trieste, and the Northwestern
University of Chicago (NW).
Technology-related research area
Carbon nanotubes and conductive polymers composites for high performance, moldable electrodes for in-vivo neural recordings
- The aim of the research is to develop a new class of
in-vivo neural recording electrodes that do not rely only on metals or
silicon based materials, opening the way to the realization of long term
neuro-electric interfaces. Particular attention is devoted to significantly
improve the signal-to-noise ratio, to explore the possibility of epicortical
mid-impedance recordings, to improve the biocompatibility of implants and
therefore their temporal stability.
By exploiting the ability to control down to the molecular level the
manifold properties exhibited by polymers (such as variable mechanical
stiffness, actuation, electrical conductivity and bio-functionalization)
together with the outstanding electrical and mechanical properties of carbon
nanotubes, we envisage a totally new way of manufacturing nano, micro and
macro scale neural probes with very low anatomical impact and high
efficiency. In the long run, an all "organic" implantable system (i.e.
including signal conditioning) can be foreseen.
Microelectronics for signal conditioning and processing
of bidirectional brain signals
- In an effort to create fully implantable neural
recording/stimulating devices, scientists of this lab, in collaboration with
the "Politecnico di Milano", are interested in combining multi-electrode
arrays with integrated complementary metal-oxide-semiconductor (CMOS)
electronics.
Some Application-Specific-Integration-Circuits (ASICs) are being designed
within this lab, in order to be used in the development of
brain-machine-interfaces. A first prototype, comprising 8 low-power and
low-noise neural signal amplifiers was designed and successfully tested
in-vivo with rats. Two distinct solutions comprising respectively 16 and 64
channels, with on-chip A to D conversion and spike detection are currently
under test. Wireless communication solutions, as well as stimulation
circuits to provide sensory feedbacks are currently subject of study
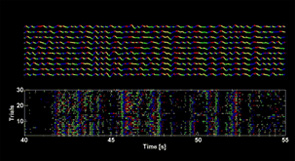
Optimal and stable extraction of information from brain
signals (local fields and action potentials)
- It is widely believed that the biggest bottleneck in the
construction of Brain-Machine Interfaces comes from the relatively little
amount of control and information about intended actions or relevant sensory
variables that we are able to extract from the extracellular neural signals
recorded from the brain. The research of the Brain Signal Analysis
Laboratory aims at enlarging this bottleneck, by devising optimal ways to
extract as much information as possible from brain recordings. This is aims
is pursued by 1) designing mathematical methods, mainly based on information
theory and Bayesian decoding (Quian Quiroga and Panzeri, Nature Reviews
Neuroscience 2009) that can extract as much information about external
correlates as possible from the signals that can be obtained from
extracellular electrodes (such as Local Field Potentials (LFPs), or action
potentials (APs) 2) by applying these methods to real data to learn more on
how the activity of sensory and motor cortices conveys information.
Examples of recordings of slow Local Field Potential waves (top panel) and
of action potentials (shown as dots in the bottom panel) in primary visual
cortex during the presentation of color movies. In both panels, each line
plots the response to different repetitions of the same movie.
Neurophysiology-related research area
Mapping of motor/premotor functions in individual brains
- Recordings of neuronal activity (individual neurons,
evoked potentials, local field potentials) are generally performed on
cerebral regions identified according to anatomical criteria, the main ones
being the configuration of the cerebral sulci (for the cortex) and the
stereotaxic position (for the subcortical nuclei). On the other hand, the
recent introduction of functional magnetic resonance makes it now possible
to localize on single individuals the cerebral regions that become active
during particular motor, perceptual or cognitive tasks.
In neurosurgery patients, during the electrophysiological characterization
of the brain tissue surrounding a cancer, the association of fMRI
(pre-operative) with recording of nerve activity (intraoperative) opens up
immense research possibilities as it makes it possible to record neurons
exactly where cerebral activation is known to be present. This provides on
the one hand precious information to the surgeon about the functional
borders of the tumor, on the other hand, the large number of case histories
and the ubiquitarian nature of the gliomes (the type of tumors more often
requiring such a functional mapping) allow numerous recordings to be made in
motor and premotor regions every year.
This sub-project is done in collaboration with the Neurosurgery Department
of the Udine Hospital and the Section of Human Physiology of the University
of Ferrara.
Study and implementation of in-vivo techniques for
bidirectional communication with the nervous system
- The main idea of a Brain Machine Interface consists in
extracting neural signals directly from the brain and use them to control
external devices. In the framwork of building neuralprostheses this
technique could be useful to better understand how the brain processes the
sensory information coming from the environment and uses it to build motor
commands. To reach this result a crucial point is to develop a BMI real-time
system to create a bidirectional communication channel with the nervous
system. In our lab we are implementing in-vivo techniques using
multielectrode microwires arrays chronically implanted in the cortex of
awake rodents. These techniques permit to record the neural activity from
the cortex while the animal is behaving and simoultaneously to deliver
Intracortical Micro Stimulation (ICMS) patterns providing an artificial
input in a closed-loop system.
Brain Imaging Lab
- The study of the functional response of the motor
cortex during the programming, execution and mental representation of
voluntary movements is of great relevance; given the high integration of the
visuo-motor, sensory feedback and proprioceptive systems, it is important to
be able to evaluate it in conditions that closely approximate the real.
The main goal of the activity here is to develop an MR machine that could be
used to record functional data from subjects maintaining an an erect stance
(at least for the trunk), and with sufficient limb freedom to afford the
execution of simple motor tasks.
The main effort in this activity is devoted to the finalization of the
project of the magnet.
A relevant part of the project deals with the optimization of the structure,
to make it easier to transfer to cost sensitive applications such as
conventional clinical imaging; another significant area is represented by
the field compensation techniques that will merge with the design of the
gradient coils.
In parallel to the fMRI scanner design a close eye will be kept on the
development of the Near Infrared Spectroscopy (NIRS). It is a complementary
technique that offers, with respect to fMRI, a worse spatial resolution but
presents the advantage of a much lighter equipment and could become, once
fully developed, a valuable complement to MRI.
Tissue Engineering Lab
- One of the IIT main technological goals is to move
ahead from traditional humanoids with mechanical hands and legs (hard-bodied
systems) toward next generation hybrid systems realized with soft materials,
artificial muscles, tendons, growing tissues, bio-sensors (soft-bodied
systems). This ambitious goal requires a deep investigation of soft,
functional, anisotropic materials mimicking our skin, tendons and bones but
also development of self-repairing, evolvable materials. By endowing such
materials with appropriate biocompatible and functional properties, highly
efficient interfaces between biological systems and artificial devices may
be created, allowing the development of innovative prosthetic devices.
To this end a Tissue Engineering Laboratory is established in Morego
bringing together researchers from the IIT Central Labs and IIT Network with
the common aim of interfacing biological systems to artificial devices.
Topics addressed:- Formulating nanobiomaterial scaffolds
- Understanding and controlling cellular response: 3D organization
- Bio-reactor based tissue engineering and testing
- Robotic Prostheses development
- Coupling of structural elements of artificial limbs with bone tissue